Note: Image adopted from UCL Faculty of Mathematical and Physical Sciences at http://www.ucl.ac.uk/maps-faculty/potw/potw/potw1313
Today it is hard to imagine a world where smartphones, laptops, smartwatches, and electric vehicles are not rechargeable. This indispensable attribute is thanks to the lithium-ion battery (LIB) which was first commercialized by Sony and Asahi Kasei in 1991 [i]. Our demand for LIBs does not seem to decrease or stagnate as more personal devices and especially electric vehicles are introduced to the market. The global electric vehicle stock increased exponentially from 1.18 million in 2016 to 4.79 million in 2019 [ii] and the trend is expected to continue. Therefore, continuous development and improvement of LIBs are of great importance.
Content
– The working principle of lithium-ion batteries
– Nanostructured anode and cathode materials
– Outlook at commercial products and the successor to lithium-ion batteries
Working Principle of Lithium-Ion Batteries
The common present-day LIBs are similar to the ones commercialized in 1991 and are composed of two electrodes, a separator, and a liquid electrolyte, as seen in the figure below.
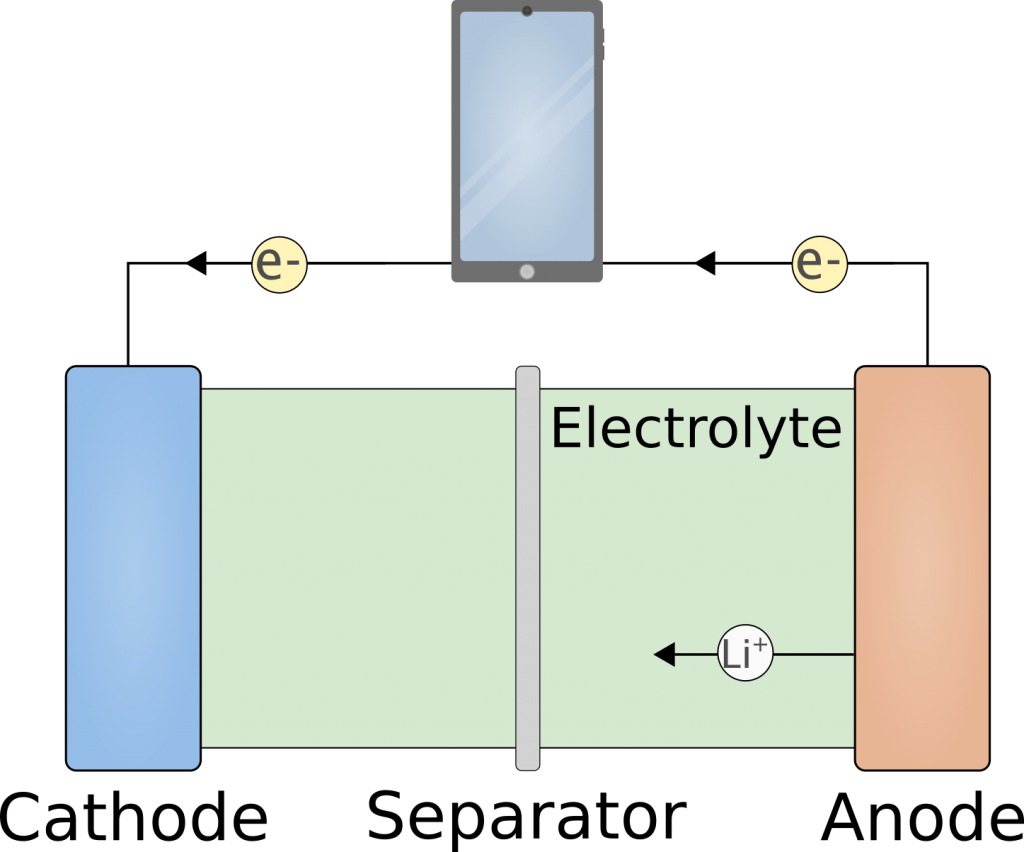
The positive electrode (cathode) is commonly a lithium metal oxide such as LiCoO2 while the negative electrode (anode) is made from graphite. When the LIB is charged the lithium ions shuttle from the cathode to the anode while electrons are moved away from the cathode and supplied at the anode.
When the LIB is discharged the process is reversed and the lithium ions shuttle internally from the anode to the cathode where they intercalate. The electrons move externally from the anode to the cathode and as they move, they can be used to power a device such as a smartphone. The capacity of the LIB is hence limited by the amount of lithium that can be stored in the cathode and the anode structure. It can be increased by enlarging the batteries but since this is often not desired, much attention is given to increase the capacity per kilogram of battery i.e. the specific capacity.
This can be done by creating new cathode and anode compounds which can accommodate more lithium per kilogram. However, this often comes with great challenges e.g. silicon as an anode material has about ten times higher specific capacity compared to graphite but it suffers from drastic volume changes during charge/discharge [iii]. Another way to increase the specific capacity is to optimize the structure of the anode and cathode material such that a larger portion of it participates in the intercalation process.
Nanostructured Anode and Cathode
The anode in commercial LIBs is based on graphite which is the same material used in pencils. It is used due to its price, availability, and relatively good theoretical specific capacity of 372 mAh g-1 [iv]. Despite the success graphite has had, it is still relevant to improve the anode in terms of e.g. the specific capacity. Some of the promising nanostructured carbon allotropes that could be used instead are: Carbon nanotubes (CNTs), graphene nanosheets, and carbon with nanosized pores. [iv]
It has been demonstrated that the CNTs, which are rolled sheets of graphene, can accommodate lithium ions both on the inside and outside, and that they have excellent electronic conductivity. It was also reported that by introducing defects into the CNTs the capacity improved. [iv]
Another option is graphene nanosheets, which can be prepared by exfoliation from bulk graphite since it is composed of stacked layers of graphene. Similar to the CNTs the surface area is greater compared to graphite and since both the plane and the edge of the nanosheets can accommodate lithium ions the theoretical specific capacity can reach 744 mAh g-1. Furthermore, it can be increased to over 1000 mAh g-1 by introducing defects. One of the difficulties with graphene is its low density and hence it requires a larger anode volume which ultimately leads to a larger battery. Another difficulty is that it participates in undesired reactions leading to irreversible loss of capacity. [iv]
A third alternative is to create nanosized pores in carbonaceous materials. One approach is to make hollow spheres with a double-shelled nanostructure which increases the surface area and thus the specific capacity. The specific capacity for such a structure has been reported to reach 920 mAh g-1. However, the increased surface area inevitably results in obstacles and defects which may trap lithium ions which in turn causes an irreversible capacity loss. [iv]
While the anode material is based on carbon, the cathode material in common commercial LIBs is in general either a lithium metal oxide such as LiCoO2 (which was introduced by J. B. Goodenough and was the first commercial cathode) and LiNiMnCoO2, or a phosphate-based compound such as LiFePO4. These cathode materials are used today along with multiple other types depending on the desired voltage and working environment. LiCoO2 and LiNiMnCoO2 are some of the most common cathode materials due to their great specific capacity of about 150 mAh g-1, and good cyclability. They, however, rely on cobalt which is expensive, toxic, and poses both environmental and health concerns due to the conditions in which it is mined and extracted.
In order to lower the amount of cobalt and increase the specific capacity, it has been proposed to substitute some of the cobalt with manganese (Mn) and Nickel (Ni). It has been reported that a nanosheet structure of LiNi1/3Co1/3Mn1/3O2 with width and thickness of 0.7-1.5 µm and 10-100 nm, respectively, delivered an increased specific capacity of 193 mAh g-1. This is due to the high surface area of the nanosheets. [iv]
Outlook
Many of the nanostructured cathode and anode materials are still in the research phase but some have already been commercialized. An example is a LiFePO4/Graphite based LIB from LithiumWerks which utilizes their trademarked Lithium Nanophosphate technology [v]. Another example is the porous carbon material usable in both the anode and cathode developed by Graphene Batteries [vi].
Lithium-Ion batteries have and will be important for our transition to green energy but as our demand for inter alia higher capacities and higher power output keeps increasing we may have to look towards other materials for both the anode, cathode, and the electrolyte. This will give us new problems which we have to overcome, perhaps through nanostructuring?
If you’d like to learn more about nanotechnology, please subscribe to our newsletter and stay tuned for upcoming posts.
References
[i] George Crabtree, Elizabeth Kócs, and Lynn Trahey, ”The energy-storage frontier: Lithium-ion batteries and beyond”, MRS Bulletin, 40, 2015, pp. 1067-1078
https://www.cambridge.org/core/journals/mrs-bulletin/article/energystorage-frontier-lithiumion-batteries-and-beyond/A0CE1F1D2F344EB6B362DA3C29DC2BD1/core-reader
[ii] International Energy Agency, “Global EV Outlook 2020 – Entering the decade of electric drive?”, Technology report, 2020
https://www.iea.org/reports/global-ev-outlook-2020
[iii] Xuefeng Song, Xiaobing Wang, Zhuang Sun, Peng Zhang, and Lian Gao, “Recent Developments in Silicon Anode Materials for High Performance Lithium-Ion Batteries”, Merck, [Accessed on: 08/11-2020]
https://www.sigmaaldrich.com/technical-documents/articles/materials-science/recent-developments-in-silicon-anode-materials.html
[iv] Wei Zhao, Woosung Choi, and Won-Sub Yoon, “Nanostructured Electrode Materials for Rechargeable Lithium-Ion Batteries”, Journal of Electrochemical Science and Technology, 11, 2020, pp. 195-219
https://www.jecst.org/journal/view.php?number=335
[v] LithiumWerks, [Accessed on: 08/11-2020]
https://lithiumwerks.com/
[vi] Graphene Batteries, [Accessed on: 08/11-2020]
https://www.graphenebatteries.no/